Current Projects
Visual System Response to Electrical Simulation
The long-term goal of this project is to investigate safe and effective retinal stimulation paradigms. We conduct experiments in animal models, including models of inherited outer retinal degeneration, and in humans with Argus II retinal implants. In animals, we use single-cell electrophysiology and calcium imaging to assess the response at cellular resolution. We also use optical coherence tomography to measure the safety of high-intensity, continuous stimulation. To assess visual receptive fields, we measure visual cortex responses in small animals. Human testing focuses on the shape and other perceptual characteristics of single and multi-channel stimulation.
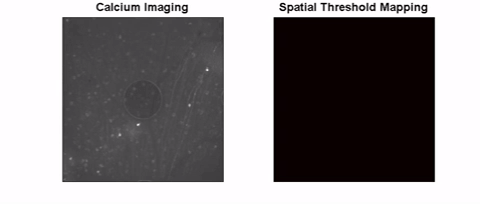
Human Functional Neuroimaging in Blindness and Sight Restoration
Visual impairment and sight restoration affect the brain in ways that are not yet fully known to us. Visual sensory deprivation in the late-blind has been shown to lead to neural reorganization and processing of other sensory modalities, e.g. auditory and tactile, in the visual cortex, and this reorganization may limit the effectiveness of therapies that restore vision. We use functional MRI to shed light on the considerable knowledge gap that precludes our understanding of these mechanisms. We extract cortical functional connectivity to investigate local and network-wide brain alterations in response to blindness and sight restoration, thus, gaining a better understanding of how vision deprivation affects the interaction and organization of the brain.
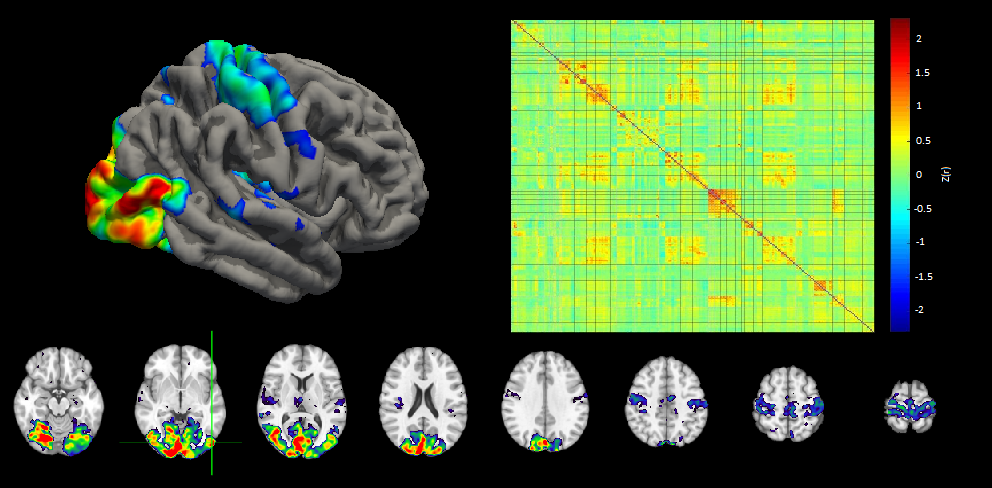
Neuroplasticity’s Role in Vision Replacement
The brain conforms to external stimuli and this is especially the case with altered sensory information. With neuroplasticity comes the concern of undesired, irreversible changes in the adult brain. Based on the findings that efficacy of cochlear implants negatively correlates with cross-modal plasticity intensity, vision replacement technology efficacy may also be impacted by the brain’s inability to receive artificial sensory information. Clinical studies have revealed that extended retinal prosthesis use increases excitability of the primary visual cortex. We seek to answer if the brain is ever locked out of vision replacement stimulation, and how the brain’s long-term reaction to electrical sensory stimulation can inform improvements to vision replacement technology device design and to use training.
Materials for Bioelectronic Implants
Widefield bilayer array
Current retinal prostheses are mainly made of rigid material with non-fitting curvature, and have a lack of coverage of peripheral vision. We propose to design a wide-field epiretinal prosthesis with soft materials and a fitting curvature. Currently, we have demonstrated a hydrogel-PDMS bilayer structure that curves in a predictable manner when immersed in water. Initial monomer concentration is directly related to the resulting curvature. For the next step, we will fabricate the epiretinal prosthesis using PDMS for the multielectrode array substrate and insulation layers, and polyacrylamide hydrogel as the shape-changing layer. Finally, the conducting lines will be gold, with additional adhesion layers as necessary. Electrodes can be electrodeposited platinum or platinum-iridium.
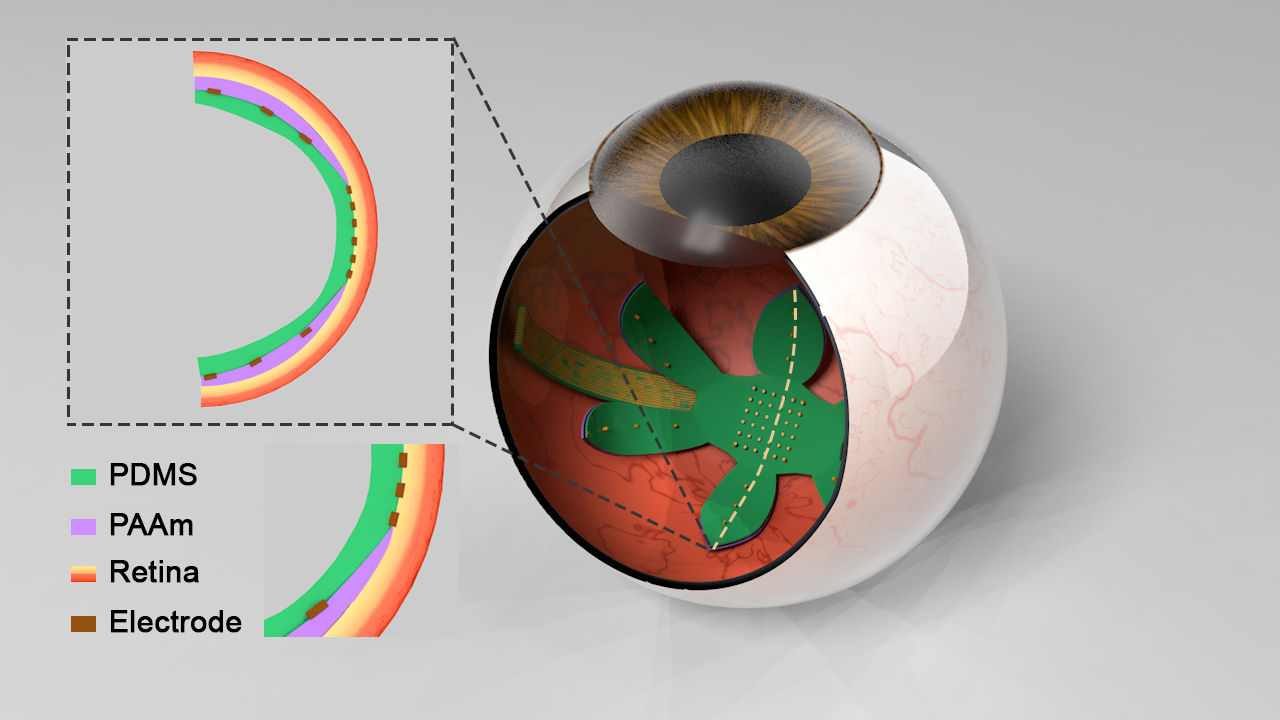
PtIr coating to enhance electric and mechanical stability of microelectrodes
Next-generation brain-machine interfaces may use thin-film metals or non-metallic materials (e.g. carbon) for electrical connection to the brain. However, thin-films are not robust to electrical stimulation and some materials do not form a low-impedance electrode interface with neural tissue. We developed a Platinum – Iridium (PtIr) electrodeposition process as an electrode surface roughing method for different microelectrode arrays (planar and circular shape) to enhance their electrical and mechanical stability. For in-vitro characterization, we perform electrochemical impedance measurements (EIS), cyclic voltammetry, and we use spectroscopy imaging (SEM, TEM, STEM) to analyze composition and morphology at both the surface and within the PtIr film. The PtIr coating allows decreasing the microelectrodes impedance up to 90 % at 1 kHz on different seeding layers such as gold and carbon. Chronic in-vitro electric pulsing tests have been done on carbon fiber microelectrodes to test the stability of the film in response to biphasic electric pulses with a charge density of 1 mC/cm2 and duration up to 300 µs. No structural modifications were noticed after 13 million electric pulses in 12 hours and voltage transients recorded before and after the 13 million pulses were stable. Currently, the performance of PtIr-coated carbon fiber microelectrodes is being tested by in-vitro retina stimulation for calcium imaging expression to assess the response at the cellular level.
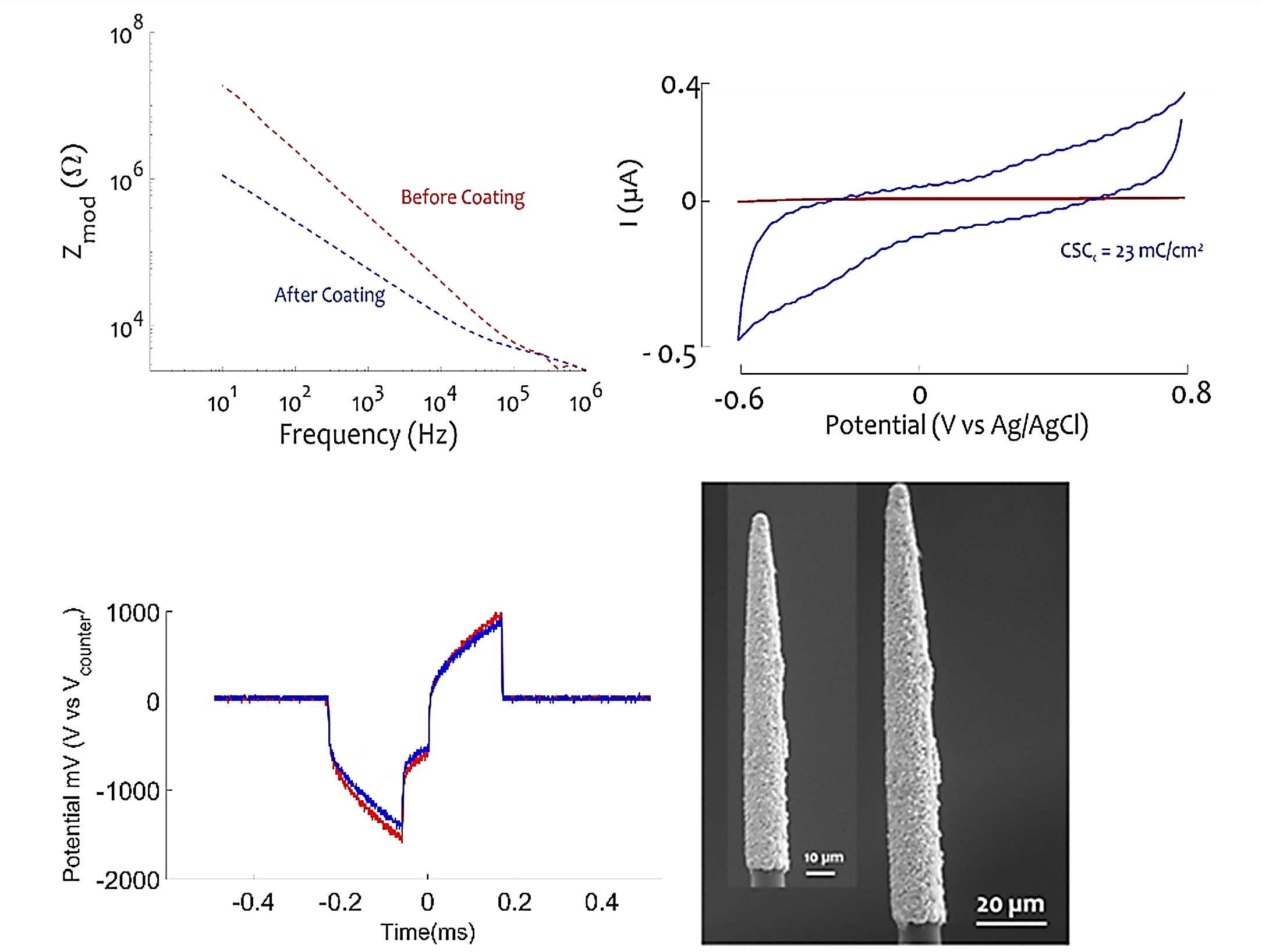
EIS (top left) and CV (top right) of CF before and after PtIr deposition. (Bottom left) voltage transients for CF-PtIr before (red) and after (blue) 13 million current pulses in 12 hours. (0.17 ms, 70 µA, ~ 1 mC/cm2). (bottom right) SEM before and after pulsing.
(Disclosure – Dr. Weiland has a financial interest in Platinum Group Coatings, which is commercializing this material).
Wearable Computer Vision Systems for Blind Navigation
Our team is assessing the ability of computer vision programs to interpret a scene in real time and use this information to guide a blind person as they search for an object and/or navigate through a neighborhood. We demonstrated that blind people can use simple verbal or vibrotactile cues to direct them to complete reach and grasp as well as navigation tasks.
Patient-specific models to optimize retinal stimulation
Retinal prosthesis performance is limited by the variability of elicited phosphenes. The stimulating electrode’s position with respect to retinal ganglion cells (RGCs) affects both perceptual threshold and phosphene shape. We are developing a computational modeling framework that incorporates patient-specific anatomy and electrode location to investigate RGC activation and predict inter-electrode differences for retinal prosthesis users. We use ocular imaging (OCT and ultrasound) to build three-dimensional finite element models. To predict the neural response to stimulation, we couple electric fields (calculated in COMSOL) with multi-compartment cable models of RGCs (solved in NEURON). In silico results are validated with human subject data. Our patient-specific retinal stimulation models allow us to quantitatively predict retinal activation and better explain the phosphene variations that occur across patients and electrodes. We use our models to optimize electrical stimulation parameters in order to improve the quality of artificial vision.
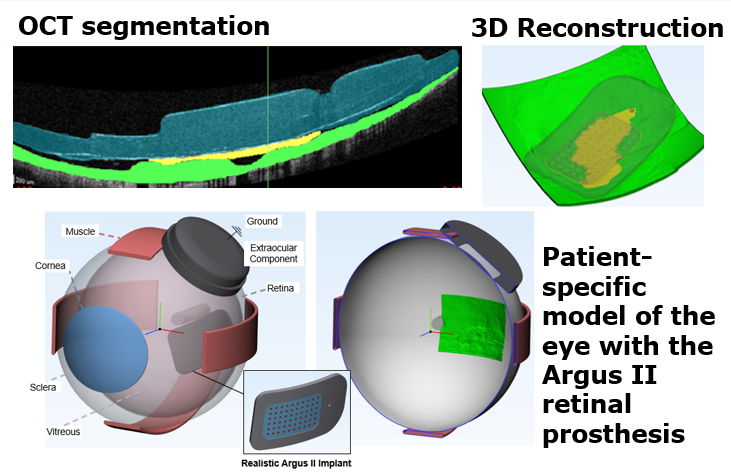
Retinal Electrophysiology
This study focuses on the effect of GABA on the mice retinal ganglion cells (RGCs) to identify the regulatory role of amacrine cells in RGCs. RGCs were classified into ON, OFF, and ON-OFF types using 10-second light exposure. Electrical stimulation pulses were applied via an epiretinal electrode (platinum, 75 micron diameter). Pulses were 0.5 ms, cathodic first, biphasic, with a train of 20 pulses delivered at 2 pulses/second. To obtain response data, pulse amplitude was increased in steps. Then the number of pulses that evoked spikes was counted and threshold was set at the 50% response rate. No difference was noted in threshold based on cell type. After administration of picrotoxin (100 mM), threshold decreased (control: 73.6±15.02mA; 100 mM picrotoxin: 62.36±13.95 mA, n=25, p=0.02782). RGCs spontaneous spikes rate increased after picrotoxin (control: 13.16±3.85 spikes/s; 100 mM picrotoxin: 25.27±4.65 spikes/s, n=24, p=8.581×10-5). These implied that blocking amacrine cells’ GABAa receptor increased the excitability of RGCs as reflected by decreases in action potential threshold.

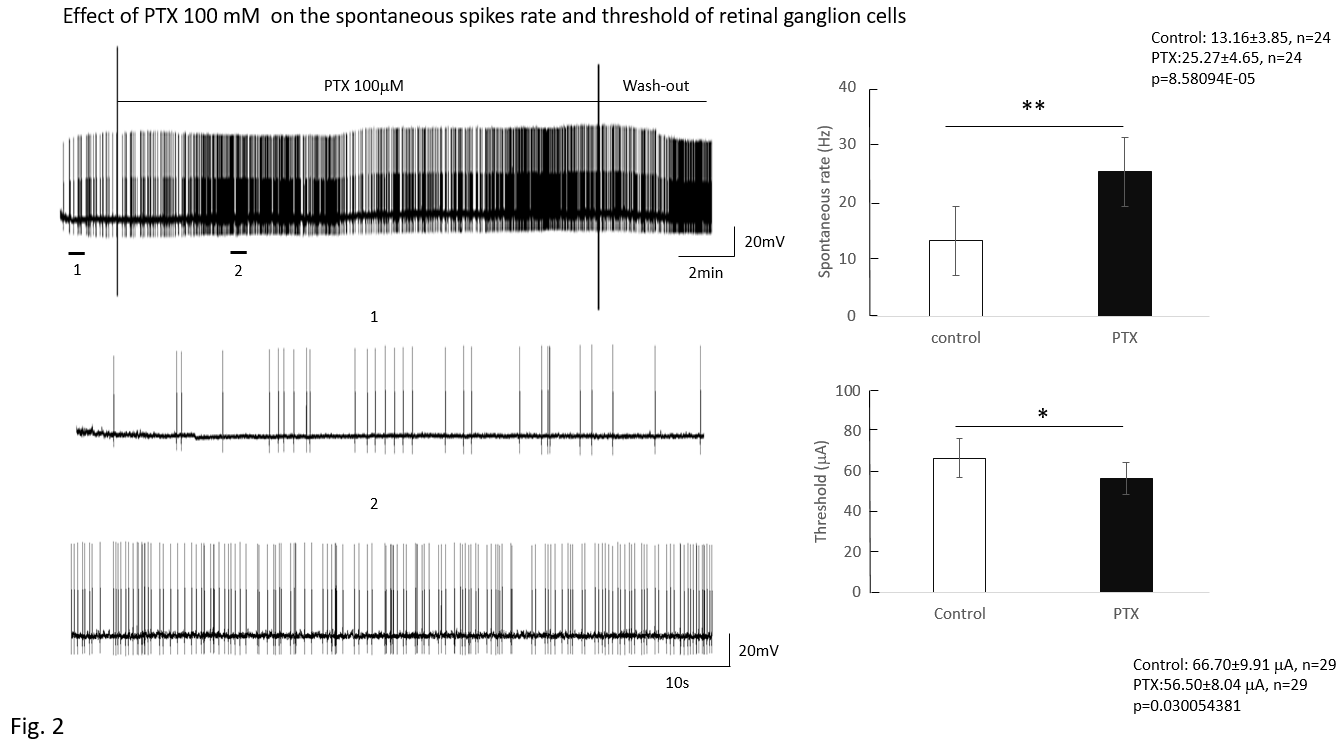